Photophysics and electronics of hybrid materials for energy-related applications
The TUM-IAS Fischer Fellowship allows for building a multidisciplinary energy-related program between the Shustova (USA) and Fischer (TUM) groups, resulting in 24 publications in the top chemistry journals, including Nature Communications and Nature Chemistry. As a result of productive collaboration, Prof. Shustova was selected as a recipient of the Bessel Research Award from the Humboldt Foundation.
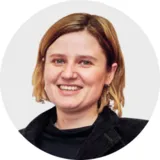
Focus Group: Photophysics and Electronic Structure of Metal-Organic Frameworks
Prof. Natalia Shustova (University of South Carolina), Alumna Hans Fischer Fellow | Johanna Haimerl (TUM), Doctoral Candidate | Host: Prof. Roland Fischer (TUM)
Figure 1
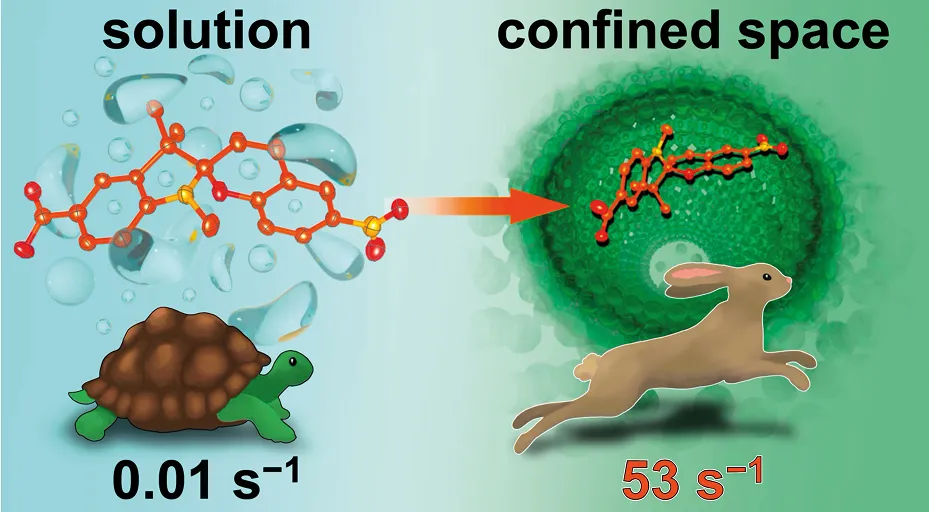
Breakthroughs in hybrid material performance for energy-related applications
Outcomes of the fruitful interdisciplinary program between the Fischer and Shustova research groups built due to the TUM-IAS Hans Fischer Fellowship resulted in 24 publications, as well as the mentorship of the graduate student Johanna Haimerl, whose graduation is expected in 2025. The results acquired due to the TUM-IAS Fellowship laid the groundwork for Prof. Shustova receiving the following awards during the Fellowship period: the competitive Friedrich Wilhelm Bessel Research Award from the Alexander von Humboldt Foundation and the Russell Research Award.
Development of on-demand-activated drug delivery platforms, photochromic heterogeneous catalysts, molecular motors, recyclable and healable materials, artificial muscles, multilevel anticounterfeiting and information encryption systems, and tailorable supercapacitors fully relies on fast changes between distinct states occurring in the solid state. Therefore, as a part of the Fellowship, we studied rapid switching between two (or more) discrete states in the solid state, which is a cornerstone for the technological development of devices based on stimuli-responsive materials. It could be anticipated that the forthcoming breakthroughs in artificial muscles, supercapacitors, and especially optoelectronics would critically hinge on substantial improvements in existing switching rates of stimuli-responsive building blocks. However, even in solution, where the molecules exhibit more degrees of freedom in comparison with the solid state, the rates of such processes are typically varied from 10−5 to 100 s–1 using light as an external stimulus. By utilizing solvent polarity and viscosity as variables, the speed of the photoisomerization process can be enhanced, but typically within one order of magnitude. In the solid state, switching between distinct states is usually further constrained compared to solutions due to close packing, π-π stacking, or hydrogen-bonding interactions, especially for molecules for which isomerization is accompanied by large structural transformations or formation of zwitterionic species (e.g., spiropyran).
Figure 2
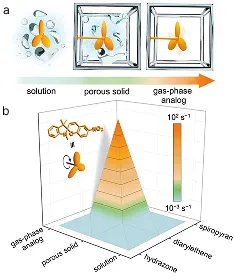
speed limit, a critical aspect in the material design, achieved through the integration of a photochromic molecule (orange propeller) within the evacuated porous hybrid materials (a), a novel concept developed due to the Shustova-Fischer collaboration. (b) Comparison of the achieved isomerization rate constants (depicted by the orange color) with the values previously reported in the literature (shown in blue and green colors). Image: 2023 Nature Communications
Due to fruitful collaborations between the Shustova and Fischer groups, we were able to report a breakthrough in the isomerization speed limit of photochromic molecules on the example of sterically-demanding spiropyran derivatives, achieved through the employment of a conceptually novel strategy, allowing for not only precise control of photoswitch environment (e.g., solvent-free) but also environment tunability due to matrix modularity (Figs. 1 and 2). Furthermore, this approach led to a drastic enhancement of photoswitch isomerization ability in the solid state, addressing challenges associated with limited photoisomerization due to strong intermolecular interactions typically pronounced in the solid state and impeding the development of stimuli-responsive materials.
To the best of our knowledge, such a rapid response observed in the solid state, through the integration of spiropyran derivatives into the confined solvent-free space of rigid frameworks, has no analogs for these classes of photoswitches either in solution or in the solid state. While slight enhancements in photoisomerization rate could be anticipated and achieved using organic solvent as a parameter, the presented conceptually different approach, realized through construction of the spiropyran environment, results in ~1,000 times switching enhancement even in the solid state compared to its behavior in solution, setting a record in the field of photochromic compounds (Fig. 1). Using spectroscopic analysis in combination with photophysical measurements and theoretical modeling, we shed light on the mechanism of possible rapid photoisomerization. Thus, the strategic design of light-responsive materials has resulted in rate enhancement that surpasses the preconceived “speed limit” for the commonly used classes of photoswitches, including but not limited to spiropyran-, diarylethene-, and hydrazone-based photochromic derivatives (Fig. 2). Furthermore, the approach we developed allows for the integration of more than one type of photochromic molecule within the same platform, providing access to the development of solid-state materials with a very broad dynamic range of photoisomerization rates (Fig. 3).
To summarize one of the outcomes achieved during the Fellowship period, the presented conceptual approach, realized through the construction of the spiropyran environment, sets the record in the field of photochromic materials. Moreover, the integration of two distinct photochromic moieties in the same framework provided access to a dynamic range of rates as well as complementary switching in the material’s optical profile, uncovering a previously inaccessible pathway for interstate rapid photoisomerization. This concept is a critical component for the development of a forthcoming generation of materials, including recyclable and healable systems, artificial muscles, photochromic heterogeneous catalysts, and tailorable supercapacitors that all rely on the fundamental concept of rapid switching between two or more discrete forms in the solid state.
Figure 3
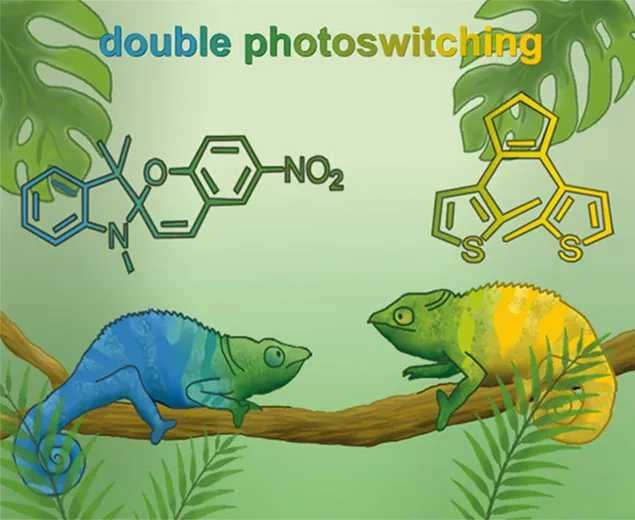
Selected publications
- Stanley, P. M., Haimerl, J., Shustova, N. B., Fischer, R. A. & Warnan, J. Merging Molecular Catalysts and Metal-Organic Frameworks for Photocatalytic Fuel Production. Nat. Chem. 14, 1342–1356 (2022).
- Thaggard, G. C. et al. Breaking the Photoswitch Speed Limit. Nat. Commun. 14, 7556 (2023).
- Wilson, G. R. et al. Cooperative and Orthogonal Switching in the Solid State Enabled by Metal-Organic Framework Confinement Leading to a Thermo-Photochromic Platform. Angew. Chem. Int. Ed. 62, e202308715 (2023).
- Thaggard, G. C. et al. Metal-Photoswitch Friendship: From Photochromic Complexes to Functional Materials. J. Am. Chem. Soc. 144, 23249–23263 (2022).
- Thaggard, G. C., Haimerl, J., Fischer, R. A., Park, K. C. & Shustova, N. B. Traffic Lights for Catalysis: Stimuli-Responsive Molecular and Extended Catalytic Systems. Angew. Chem. Int. Ed. 62, e202302859 (2023).