Decarbonizing power generation with hydrogen gas turbines
To fulfill the goals of the Paris Agreement, the share of power generation from renewable sources will increase rapidly, resulting in fluctuations in electric power output. A reliable way to compensate for this volatility is the use of gas turbines operated on CO2-free fuels. The work of the Focus Group tackles one of the major challenges in the development of clean combustion technologies: combustion stability.
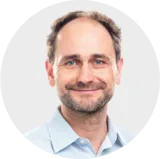
Focus Group: Data-Driven Dynamical Systems Analysis in Fluid Mechanics
Prof. Mirko R. Bothien (Ansaldo Energia Switzerland, now Zurich University of Applied Sciences and NTNU Trondheim), Alumnus Rudolf Diesel Industry Fellow | Host: Prof. Thomas Sattelmayer (TUM)
(Image: Zurich University of Applied Sciences)
Introduction
By 2050, net CO2 emissions must be reduced to zero to fulfill the goals of the Paris Agreement. To achieve this, the share of power generation from non-dispatchable renewable energy sources (RES), i.e., wind and solar, will increase rapidly. As a result, fluctuations in electric power output from these RES will increase in amplitude and impact, requiring robust and rapid grid-stabilization measures. A reliable way to compensate for this (seasonal) volatility is the use of gas turbines operated on alternative, CO2-free fuels that can stabilize the grid and provide dispatchable power. Alternative fuels such as hydrogen and ammonia are optimally suited for seasonal, large-scale chemical storage in periods of overproduction from non-dispatchable RES and can be burned in gas turbines at times when RES do not satisfy the need.
Most gas turbine manufacturers are using axially staged or sequential combustion systems in their newest engines. Consisting of two combustion chambers arranged in series, this concept is the method of choice for alternative fuels because of its superior fuel flexibility obtained by different flame stabilization mechanisms. This is especially important for the transition phase from 2030 onward, in which the supply of alternative fuels will be unsteady, and the engines will need to handle and seamlessly switch between a broad variety of different fuel compositions.
Besides fulfilling stringent emission regulations, one of the major challenges in the development of any clean combustion technology is to ensure dynamic stability, also referred to as thermoacoustic stability. A system can get thermoacoustically unstable due to a constructive coupling of fluctuating heat release with the system’s acoustics, severely limiting engine operation. Up to now, the thermoacoustics of the second stage combustor have been studied much less than those of the first stage. Flame stabilization in the second stage is achieved by autoignition. Hence, the involved physics and methods substantially differ from the well-studied propagation-stabilized first stage flames. The work in the Focus Group targets better understanding and modeling of the thermoacoustics of autoignition flames, making it possible to identify and solve instability issues in an early design stage.
Project results
Funding for two doctoral candidates was successfully secured from the German Science Foundation (High-Frequency Flame-Acoustic Interaction Mechanisms in Reheat Flames, Jonathan McClure) and the Research Council of Norway (Dynamic Behaviour of Hydrogen Flames in Sequential Combustion Systems, Florian Franke), making it possible to perform the research. In the following, results of the former project from Jonathan McClure are presented.
To investigate the flame-acoustic coupling mechanisms driving thermoacoustic instabilities in reheat flames, the following research topics were investigated to support predictive models:
1) Development of an autoignition modulation model
2) Comparison of model results with experimental observations
3) Influence of additional superposed driving mechanisms
4) Impact of real engine conditions.
A thermoacoustic driving mechanism based on the local modulation of the autoignition delay time by acoustic perturbation was proposed and modeled numerically. Model results indicate that this mechanism represents significant driving potential when regions of high acoustic pressure amplitudes overlap with the autoignition flame. Comparison with experimental measurements confirms this as a key driver of thermoacoustic instabilities in reheat flames.
Experiments were carried out on the unique, industrial-size reheat test rig at the Technical University of Munich (Fig. 1) over a range of conditions, using synchronous acoustic and optical diagnostics to characterize the reheat flame response.
Investigation of the acoustic data from a range of experiments revealed two distinct unstable high-frequency modes. One mode experienced high-amplitude limit-cycle oscillations and was highly sensitive to fuel reactivity and power level. This indicates that the driving of the limit-cycle oscillations is strongly linked with autoignition. Investigation of the flame dynamics associated with the limit-cycle oscillations showed an axial motion of the flame in the areas near the walls where the acoustic oscillations are strongest. This motion occured in-phase with the acoustic oscillations and is illustrated in Fig. 2 (left).
By scaling a numerical estimation of the acoustic field with experimentally measured pressure amplitudes, it is possible to determine the pressure oscillations experienced by the fuel-air mixture as it is advected through the test rig. This is then used as an input for the reduced order model developed in the project to directly compare the predicted flame motion. The comparison can be seen in Fig. 2 (right) indicating that the in-phase motion predicted by the model is indeed present in experiments. Furthermore, the predicted magnitude of the ignition delay variation is approximately 70 % of that observed in experiments, suggesting that the autoignition modulation mechanism is the dominant mechanism behind the observed flame dynamics.
The project results provide important insight into the physics of autoignition flame dynamics relevant for industrial-size combustors. The findings will help in modeling and mitigating thermoacoustic instabilities in the early design stage of new combustors for alternative fuels and are a starting point to include the important effect of mean pressure level.
Figure 2
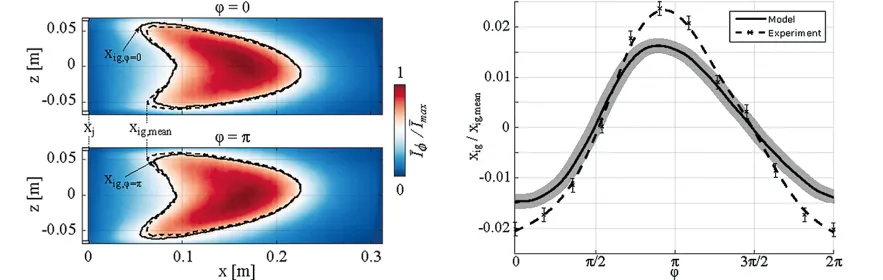
Fostering the exchange between academia and industry
The Focus Group organized the Symposium on Thermoacoustics in Combustion – Industry meets Academia (SoTiC) in 2021. A total of 170 participants attended the online symposium, with 64 papers presented, making SoTiC 2021 one of the largest – if not, the largest – event with respect to combustion dynamics so far. The symposium attracted interest from the technical and scientific community working in the field of combustion instabilities. Attendees enjoyed a week of interesting presentations on current research in the field of combustion instabilities in gas turbines and rocket engines. The format is now well established. In 2023, Mirko Bothien and Thomas Sattelmayer co-organized SoTiC at ETH Zurich in Switzerland. More than 120 thermoacousticians from 15 countries attended outstanding invited talks and 60 high-quality technical presentations followed by lively discussions. SoTiC 2025 will take place at NTNU Trondheim, Norway, where Mirko Bothien holds an Associate Professorship.
Selected publications
-
McClure, J., Bothien, M. R. & Sattelmayer, T. High-Frequency Mode Shape Dependent Flame-Acoustic Interactions in Reheat Flames. Journal of Engineering for Gas Turbines and Power 145(1), p. 011014 (2022).
-
McClure, J., Bothien, M. R. & Sattelmayer, T. Autoignition Delay Modulation by High-Frequency Thermoacoustic Oscillations in Reheat Flames. Proceedings of the Combustion Institute 39(4), pp. 4691–4700 (2022).
-
Gruber, A., Bothien, M. R., Ciani, A., Aditya, K., Chen, J. & Williams, F. Direct Numerical Simulation of hydrogen combustion at auto-ignitive conditions: ignition, stability and turbulent reaction-front velocity. Combustion and Flame 229, p. 111385 (2021).
-
Gant, F., Bunkute, B. & Bothien, M. R. Reheat flames response to entropy waves. Proceedings of the Combustion Institute 38(4), pp. 6271-6278 (2021).
-
Bothien, M. R., Ciani, A., Wood, J. P. & Fruechtel, G. Toward Decarbonized Power Generation With Gas Turbines by Using Sequential Combustion for Burning Hydrogen. Journal of Engineering for Gas Turbines and Power 141(12), p. 121013 (2019).