Skin-inspired mechanically flexible and stretchable electronic system
The Focus Group on Artificial Electronic Skin, led by Takao Someya and Gordon Cheng, aimed at the development of a novel paradigm that could lead to important advances in robotics and also enhance the quality of life. The potential impact of artificial electronic skin is enormous, as it is applicable across multiple domains including building safer robots, healthcare monitoring, entertainment, and wearable technologies to enhance our way of life.

Focus Group Artificial Electronic Skin
Prof. Takao Someya (University of Tokyo), Alumnus Hans Fischer Senior Fellow | Host: Prof. Gordon Cheng, Cognitive Systems, TUM
This research pursues the creation of an artificial electronic skin that imitates the properties of human skin using innovative fabrication techniques of printed electronics. The concept is being used to develop a network of fully printed unit-cells integrating printed sensors and specific integrated circuitry in order to mimic the human skin’s sense of touch. A large number of sensor-cells will be connected to a cell-network that determines touch information across large areas and transmits it in a manner comparable to the human nervous system. One or more cells can be connected to a processing system to interpret the sensor information and support other perception channels of a humanoid. Printed electronics technology offers inherent advantages for the development of an artificial skin: Mechanically flexible and even stretchable materials of large size can be utilized as a substrate for recently developed inks of electronic materials. These possess interesting properties, such high conductivity, high dielectric permittivity, and tunable work functions. One of the main challenges has been the integration of these sensors on large-scale area while fulfilling mechanical requirements such as stretchability and flexibility similar to that of human skin. A similar architecture may be applied on prostheses as well as for health and fitness monitoring.
As an artificial skin, we have developed porous nanomesh-type elastic conductors that can be directly attached on human skin. The conductors consist of nanofibers and nanowires (NFs/NWs) using interfacial hydrogen bonding. We simultaneously achieve high conductivity (9190 S cm−1), high stretchability (310%), and good durability (82% resistance increase after 1000 cycles of deformation at 70% tensile strain). The direct contact between the silver NWs enables the high conductivity. The synergistic effect of the layer-by-layer structure and good adhesion between the silver NWs and the polyurethane NFs enables good mechanical properties. Furthermore, without any adhesive gel or tape, the conductors can be utilized as breathable strain sensors for precise joint motion monitoring, and as breathable sensing electrodes for continuous electrophysiological signal recording. In addition, we have developed a highly durable nanofiber-reinforced metal-elastomer composite for monitoring biological information over a long period of time without any discomfort. It is made from metal fillers, an elastomeric binder matrix, and electrospun polyvinylidene fluoride (PVDF) nanofibers for enhancing both cyclic stability and conductivity. The introduction of PVDF nanofibers enhances the toughness and suppresses crack growth by providing a fiber reinforcing effect. It is found that the conductivity of a nanofiber-reinforced elastic conductor is four times greater than that of the pristine material because the silver-rich layer is self-assembled on the top surface by a filtering effect. As a result, a stretchable electrode made from nanofiber-reinforced elastic conductors and wrinkled structures has both excellent cyclic durability and high conductivity and is stretchable up to 800%. The cyclic degradation (ΔR/R0) remains at 0.56 after 5000 stretching cycles (50% strain), whereas initial conductivity and sheet resistance are 9903 S/cm and 0.047 Ω/sq, respectively. By utilizing a highly conductive and durable elastic conductor for sensor electrodes and wiring, we were able to demonstrate a skin-tight multimodal physiological sensing suit. Continuous long-term monitoring of electrocardiogram, electromyogram, and motions during weight-lifting exercises have been successfully demonstrated without significant degradation of signal quality.
Furthermore, we have succeeded in the development of nanomesh sensors that monitor finger pressure without sensory interference. Indeed, the manipulation of the finger can be monitored without disturbing the human sensation, even though our nanomesh-based pressure sensor is directly attached to the highly sensitive fingertip. It has been scientifically and quantitatively proven that the sensor-applied finger does not exhibit any statistically significant difference compared to the bare finger, even though the attachment of a super-thin (2-μm-thick) polymeric film results in a 26% increase in the grip force. The complete elimination of sensory interference has been realized by the simultaneous achievement of extreme mechanical durability (against friction/shearing force over 100 kilopascals) while keeping the sensor sufficiently soft and thin.
In close collaboration with Dr. Sae Franklin (TUM).
Figures 1 and 2
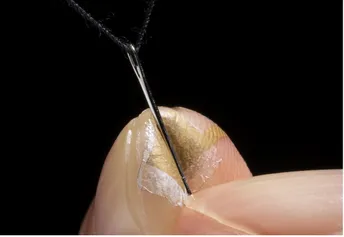
Images: Someya-Yokota-Lee Group, The University of Tokyo.
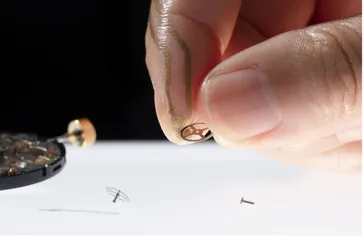