Assembling molecules using chemical reactions
The Supramolecular Chemistry Focus Group develops molecular self-assembly regulated through chemical reaction cycles. We use this method of creating molecular assemblies to synthesize materials with life-like properties; this approach might ultimately be used to synthesize life.
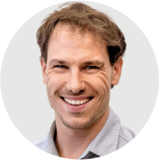
Focus Group Supramolecular Chemistry
Prof. Job Boekhoven (TUM), Alumnus Ruldolf Mößbauer Tenure Track Assistant Professor | Dr. Marta Tena-Solsona (TUM), Postdoctoral Researcher
We design molecules to bond with each other through reversible, non-covalent interactions. As a result, these molecules stack on top of each other to form larger structures, a process called self-assembly. Life could not exist without such molecular assemblies; a cell’s membrane, cytoskeletal network, and nucleus are exemplary structures. Besides biology, we also find assemblies of molecules in everyday materials such as gels, soaps, foams, and liquid crystals. The question we ask ourselves is, could we design molecules that assemble and form structures as intricate, complex, and beautiful as living ones? Could we synthesize life?
One of the reasons we cannot, yet, is because life operates out of thermodynamic equilibrium, whereas most of our knowledge in supramolecular chemistry applies to assemblies in equilibrium. Living systems, including the cell, are not thermodynamically stable but require a constant influx of energy (a cell will require nutrients to survive). As a result, most biological assemblies are controlled by energy consumption and dissipation kinetics [1]. Part of our group has concentrated on advancing the fundamental understanding of molecular self-assembly out of equilibrium. We designed molecules to self-assemble when driven by chemical reactions, which is very similar to how biology self-assembles its molecules. With that approach, we aimed to understand better why and how biology regulates structures and their function with chemical reactions. We also aimed to create materials with more life-like properties, such as materials that make autonomous decisions, for example to adapt to changes in their environment. Finally, we envision that such knowledge can help us understand better the fundamental principles of life, which could shed light on our own origins and potentially enable steps toward the synthesis of life.
We first designed a chemical reaction cycle that can act as the engine for our molecular assemblies (Figure 1A) [2]. That reaction cycle “burns” up a chemical fuel to operate. While doing this, it activates and deactivates molecules for self-assembly. In this manner, molecules can only self-assemble after being chemically activated by an energy-consuming reaction. Simultaneously, they are spontaneously deactivated by a deactivation reaction. Thus, this type of self-assembly is a non-equilibrium process (it constantly requires energy to sustain), which is fundamentally much closer to the way biology organizes its supramolecular structures.
We introduced a chemical reaction cycle that fulfills the above requirements. It uses carboxylic acid derivatives as precursors and carbodiimide-based condensing agents as fuel. The carboxylic acids are converted into metastable anhydrides (activation) when fuel is added. These anhydrides rapidly hydrolyze back to carboxylic acids (deactivation). We designed the molecules such that they would assemble into supramolecular architectures or phase-separate into droplets in the activated state. Under these conditions, the self-assembly process is kinetically controlled; that is, if a lot of fuel is present, activation outcompetes deactivation and the assemblies grow. If the system is starved of fuel, the assemblies collapse. A molecular assembly is only temporarily present if a finite amount of fuel is added. We demonstrated that this could be used as a self-erasing ink or a hydrogel that self-abolishes. In more recent work, we have shown that the assemblies can also be used to deliver drugs with tunable release profiles; we can set how rapidly and for how long a specific drug is released from its carrier. Thus we have used our approach of non-equilibrium self-assembly to control the decay profile of materials.
Figure 1
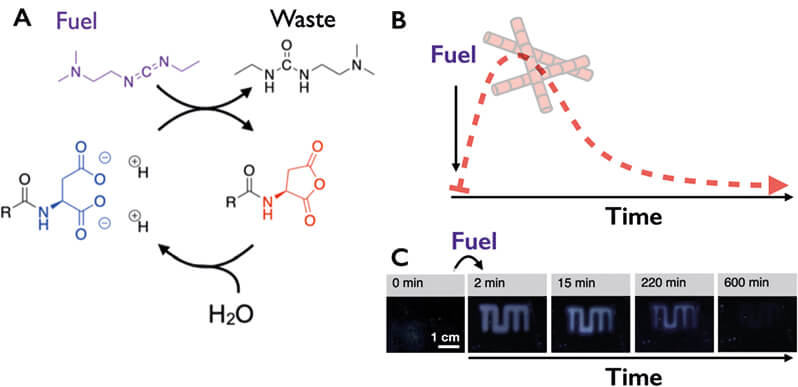
A) Scheme of the chemical reaction cycle for the fuel-driven formation of a transient product. The dicarboxylate precursor (blue) is converted into an anhydride product (red) by consumption of a carbodiimide (fuel). The aqueous anhydride is unstable and rapidly hydrolysis back to the original precursor. Because of the loss of charges when anhydride is formed, the product self-assembles upon certain concentration.
B) The result of the chemical reaction cycle is that the product and its associated properties are only temporarily present after fuel addition.
C) Using a chemical fuel as an ink, the TUM logo can be written by supramolecular assemblies. As these assemblies are fuel-dependent, the message disappears as the message runs out of fuel.
As we learned how to use our new chemical reaction cycle to drive self-assembly, we focused on bigger research questions: Can we use the transduction of chemical energy to sustain synthetic life? For that, we created a synthetic compartment that would serve as our synthetic life form. The compartments we developed are vesicles (like the cell wall), oil droplets, and complex coacervates. Each of these compartments was sustained by our chemical reaction cycle; thus each of these compartments emerged in response to energy, was sustained for some time, and then decayed when energy was depleted. Emergence, death, and compartmentalization are all hallmarks of life.
We wish to highlight one of these chemically fueled assemblies, the complex coacervate-based droplets [3]. In this work, we designed a peptide coupled to the above-mentioned chemical reaction cycle (Figure 2A and B). In its precursor state, this peptide does not interact with RNA. In contrast, in its product (activated) state, the peptide can form a complex coacervate with RNA (it can glue together the RNA). The result is that RNA-containing coacervate-based droplets emerge in response to fuel (Figure 2C). Initially, the droplets are small, but they rapidly grow due to fusion and by taking up more droplet materials (Figure 2C). When fuel is running low but not fully depleted, the droplets show signs of their evolution toward death. Large holes appear within them, so-called vacuoles (Figure 2E). In the final stage of their “life,” which coincides with the time when all fuel is depleted, these droplets lose their integrity and fall apart, though not before one final spectacle: The droplets fall apart into hundreds of tiny droplets that eventually dissolve (Figure 2F).
We believe these droplets have great potential in our design of synthetic life. First, they show some living systems traits, including emergence, death, and self-division. Moreover, these droplets have an aqueous inner compartment and can up-concentrate water-soluble molecules from their environment. Their ability to harvest nutrients from their environments opens the door to Darwinian evolution. In the next steps in our search for synthetic life, we would combine these droplets with autocatalytic reaction cycles, such that the droplets can synthesize the building blocks for their existence.
In summary, our Focus Group has made significant strides toward supramolecular chemistry out of equilibrium by introducing a simple, versatile chemical reaction cycle. The reaction cycle is now being used by other chemists worldwide. We used the cycle to demonstrate a new concept in materials science and to enable advances toward the synthesis of life.
Figure 2
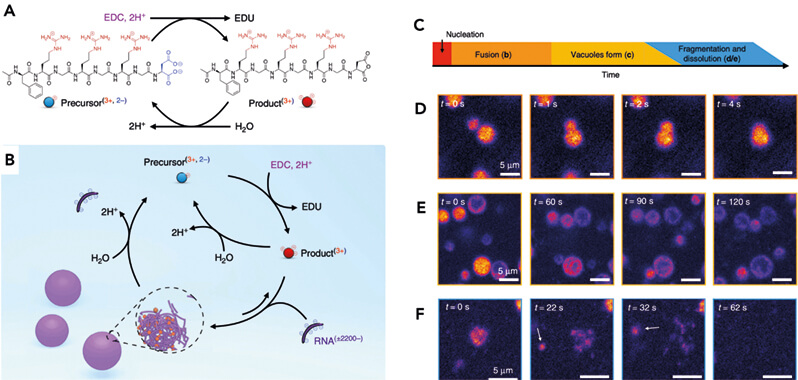
A) Molecular design of the peptide that switches affinity for RNA.
B) Schematic representation of the chemical reactions playing a role in the formation of droplets.
C) The lifetime of a chemically fueled droplet. After application of fuel, the droplets emerge. In the first minutes of their life, they rapidly fuse with other droplets. After 10 minutes, the first observation of their decay is evident, i.e., vacuoles start to form. As the system runs low on fuel, droplets start to dissolve. In some cases, dissolution results in asymmetric self-division. D–F) Confocal microscopy time lapses of the above-mentioned events.
[1]
van Rossum et al. (2017); Rieß et al. (2020).
[2]
Tena-Solsona et al. (2017).
[3]
Donau et al. (2020); Späth et al. (2021).
Selected publications
- van Rossum, S., Tena-Solsona, M., van Esch, J.H. Eelkema, R., Boekhoven, J. Dissipative Out-of-Equilibrium Assembly of Man-Made Supramolecular Materials. Chemical Society Reviews 46, 5519–5535 (2017).
- Rieß, B., Grötsch, R. K., Boekhoven, J. The Design of Dissipative Molecular Assemblies Driven by Chemical Reaction Cycles, Chem 6,3, 552–578 (2020).
- Tena-Solsona, M., Rieß, B., Grötsch, R. K., Löhrer, F. C., Wanzke, C., Käsdorf, B., Bausch, A. R., Müller-Buschbaum, P., Lieleg, O. & Boekhoven, J. Non-Equilibrium Dissipative Supramolecular Materials with a Tunable Lifetime, Nature Communications 8, 15895 (2017).
- Donau, C., Späth, F., Sosson, M., Kriebisch, B. A. K., Schnitter, F., Tena-Solsona, M., Kang, H.-S., Salibi, E., Sattler, M., Mutschler, H. & Boekhoven, J. Active Coacervate Droplets as a Model for Membraneless Organelles and Protocells. Nat Commun 11, 5167 (2020).
- Späth, F., Donau, C., Bergmann, A. M., Kränzlein, M., Synatschke, C. V., Rieger, B. & Boekhoven, J. Molecular Design of emically Fueled Peptide–Polyelectrolyte Coacervate-Based Assemblies, J. Am. Chem. Soc. 143, 4782–4789 (2021).
Full list of publications